
In recent years, cell-free protein synthesis (CFPS) has emerged as a powerful new technology, with a wide range of potential applications. Although CFPS systems have been used for decades as a research tool, it is only fairly recently that excitement has begun to build.
The promise is simple. If proteins could be synthesised outside of the constraints of a cell, the process should be much quicker (and given the lack of a cell wall), easier to control.
How well do you really know your competitors?
Access the most comprehensive Company Profiles on the market, powered by GlobalData. Save hours of research. Gain competitive edge.
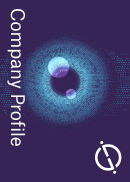
Thank you!
Your download email will arrive shortly
Not ready to buy yet? Download a free sample
We are confident about the unique quality of our Company Profiles. However, we want you to make the most beneficial decision for your business, so we offer a free sample that you can download by submitting the below form
By GlobalData“The open nature of the reaction allows the user to directly influence the biochemical systems of interest,” explains Michael Jewett, the Charles Deering McCormick Professor of Teaching Excellence and associate professor of chemical and biological engineering at NorthWestern University in Illinois. “As a result, new components can be added or synthesised, and these can be maintained at precise concentrations.”
Jewett, who co-directs Northwestern’s Center for Synthetic Biology, adds that cell-free systems enable the production of complex proteins, at concentrations that might be toxic to living cells. On top of that, processes that normally take weeks to execute can be done in days, and cell-free systems can be freeze-dried and stored safely for up to a year.
Taken together, these features could open up new biomanufacturing possibilities, not least rapid, decentralised production of medicines. While cell-based approaches require optimum conditions and expensive equipment, CFPS enables what Jewett calls a ‘just add water’ approach.
“Imagine how rapid access to vaccines in remote settings could change lives, or how new biomanufacturing paradigms suitable for use in low resource settings might promote better access to costly drugs,” he says. “Already, we and others have synthesised active antibodies, erythropoietin, and immune stimulants in less than 24 hours using cell-free methods.”
Expanding the genetic code
Jewett’s team at Northwestern, along with researchers at Yale University and the Illinois Institute of Technology, recently developed a new CFPS technique that improves the quality of the manufactured proteins.
“We were focused on developing a general, high yielding cell-free protein synthesis technology platform that was capable of expressing proteins with non-canonical amino acids for use in production of novel therapeutics,” he says.
In other words, they wanted to engineer entirely novel proteins, made from an expanded set of building blocks. This form of genetic engineering has been touted as one of the key opportunities of synthetic biology.
To take a step back, all living things – from amoebas to humans – share basically the same genetic language. All have 20 proteinogenic (protein-creating) amino acids encrypted in their genetic code, just a small fraction of the possible amino acid building blocks one could imagine.
However, if you artificially modify the genetic code, it is possible to expand the selection of amino acids used for protein creation. Over the last couple of decades, researchers have been trying to do just this, with a view to engineering new proteins with desirable characteristics. To date, more than 150 different non-canonical amino acids have been successfully incorporated into proteins, although generally just one at a time.
“A particularly exciting development surrounding cell-free technologies is the focus on expanding the range of genetically encoded chemistry into proteins,” says Jewett. “However, the task of site-specifically incorporating non-canonical amino acids into proteins with high purity and yields has presented a formidable challenge.”
Breakthroughs in the field
The problem is twofold. Firstly, all cells have a natural translation apparatus that converts genetic code into amino acids. If you want to create new proteins, you need to engineer new translational elements, which must in turn compete with the apparatus already there (typically, that isn’t very efficient).
Secondly, hijacking the genetic code can limit the fitness of the organism, causing physiological problems within the cell.
In 2013, Lajoie et al were able to overcome the first issue by genetically engineering a strain of E. coli lacking release factor 1. This was a major breakthrough in the field. However, subsequent efforts have remained limited by organism fitness. Until now.
In their latest paper, Jewett’s team developed a cell-free system using that particular E. coli strain. They were able to develop novel proteins in vivo, with multiple non-canonical amino acids, all the while avoiding viability constraints. This is another breakthrough.
“We were able to introduce 40 identical non-canonical amino acids site-specifically into proteins,” says Jewett. “To our knowledge, these are the purest polymers ever synthesised with this many site-specifically introduced non-canonical amino acids using an expanded genetic code. As a result, our work is poised to enable new classes of functional materials, and open up an entirely new area of materials chemistry research for biotechnology.”
As well as having high purity, the proteins were also high yielding.
“Our yields outperform the best expression of proteins with single or multiple non-canonical amino acids in vivo by more than 10-fold,” says Jewett. “Moreover, our yields are among the highest ever reported for CFPS systems. This suggests that long-term commercial applications of CFPS methods for discovery and manufacturing are realistic.”
Commercial uses
Already, CFPS methods are being used commercially. A number of start-ups have been established in the field, including Sutro Biopharma, which uses a cell-free system to create antibodies for treating cancer. The company is heading into human clinical trials this year. Other companies, including SynVitroBio, are using CFPS techniques to accelerate biological design in unprecedented ways.
However, it’s early days still, and many applications remain unexplored. Jewett and colleagues are currently considering how their own technique might be utilised.
“We are looking at commercial applications, especially in the synthesis of therapeutics and vaccines. We are also looking for commercial partners,” he says.
Ultimately, they want to develop new paradigms for biomanufacturing drugs on demand. This would be cheaper and speedier than anything that has come before. They are also looking to develop a freeze-dried CFPS system, which would enable distribution and storage of vaccines.
With the traditional obstacles rapidly being surmounted, CFPS systems could have an important role to play in tomorrow’s life sciences industry. A recent Economist article on the subject concluded that “for some applications at least, the utility of the biological cell may have run its course.”
As Jewett puts it: “It’s no longer a matter of if cell-free systems are going to open new avenues for biomanufacturing. It’s a matter of when. It’s really an exciting time.”