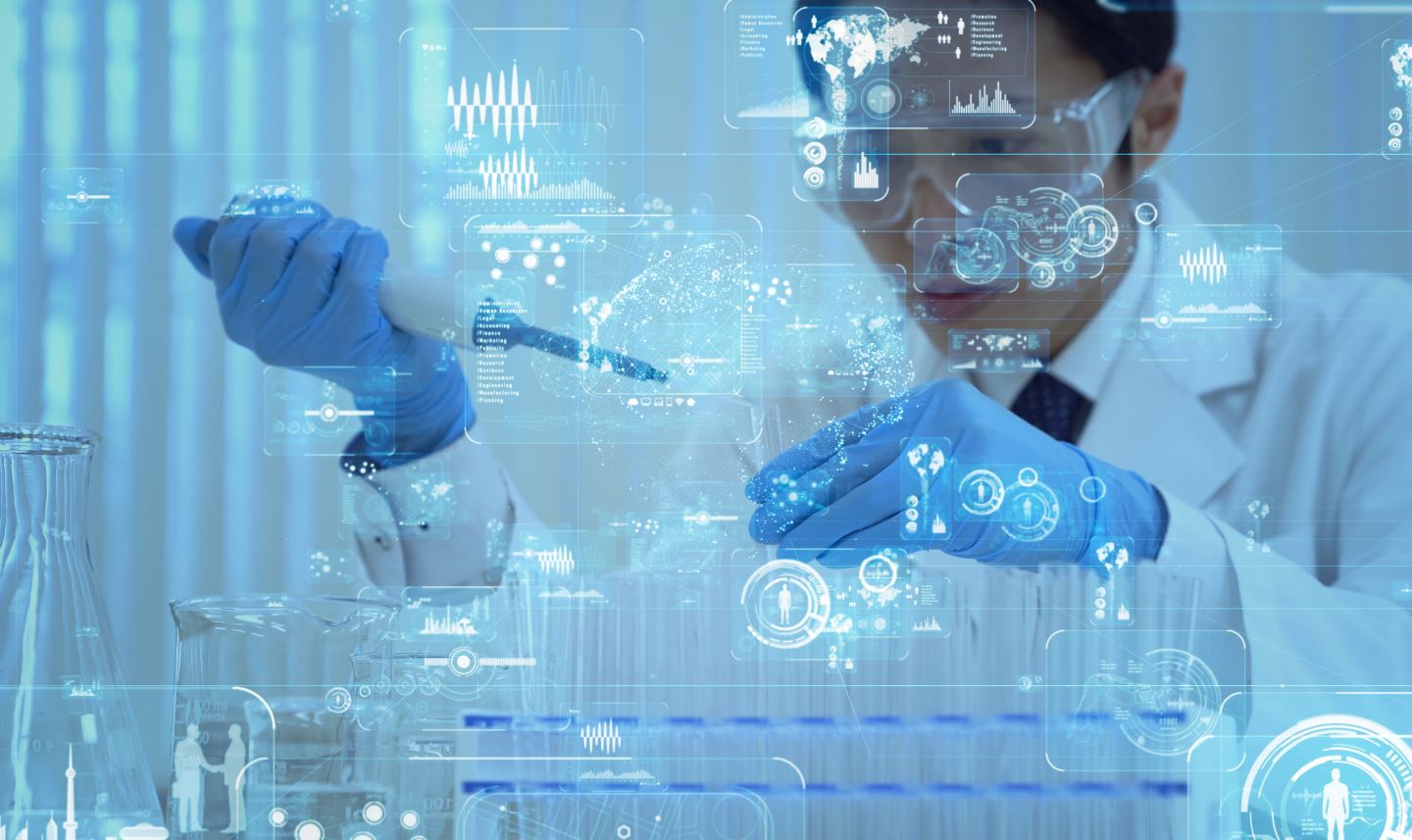
A GlobalData conducted in 2023 found that, according to 100 healthcare industry professionals, genomics was regarded as the most potentially impactful pharmaceutical trend over the course of 2024. With 12 DNA-based or mRNA-based oncology vaccines either approved or in pre-registration as of the end of 2023 according to GlobalData’s clinical drug trials database – and an additional 363 in Phase II or III development globally – the vaccine innovation landscape has never been more vibrant.
But mRNA technology is just one dimension of this innovation. Self-amplifying RNA (saRNA) is similarly promising, and if a recent surge in related publications on the NIH PubMed database is anything to go by, its potential may surpass that of its more well-known mRNA counterpart.
Compared to conventional mRNA vaccines, saRNA offers the potential for lower dosing requirements and more durable biological effects. A number of developments over the past year have highlighted the transformative potential of saRNA in therapeutics – if manufacturing methods can keep pace.
Consider developments in Japan. In November 2023, the country turbocharged saRNA’s reputation by approving ARCT-154, a novel COVID-19 vaccine developed by Arcturus Therapeutics and CSL. It marked the first instance of an saRNA-based vaccine receiving full authorisation for use as both an initial and booster vaccination for adults. Clinical trials demonstrated that ARCT-154 elicits higher levels of COVID-neutralising antibodies compared to a Pfizer/BioNTech mRNA vaccine with comparable side effects, setting a precedent for future saRNA applications. Parallel authorisation is now being sought in Europe.
In another 2023 study, the development of VLPCOV-01, an unmodified VEEV-based saRNA vaccine, showcased superior long-term immunogenicity compared to modified mRNA vaccines.[1] Subsequent reformulations of the vaccine led to VLPCOV-02, incorporating 5-methycytosines (5mC) to reduce reactogenicity without compromising immune response. Modifications like 5mC can enhance the safety profile of saRNA vaccines while maintaining or increasing their efficacy, a vital discovery that will support future biopharma research.
Another noteworthy example is a groundbreaking 2023 trial utilising TriLink’s patented CleanCap® AU analog to synthesise 26 unique saRNA variants.[2] Each of these variants substitutes a standard nucleoside with a modified counterpart. The approach led to marked improvements in cellular expression levels – sometimes up to 14-fold compared to control formulas. And, in a SARS-CoV-2 challenge study, mice immunised with saRNA incorporating TriLink’s technology and 5mC exhibited significantly improved survival rates and milder symptoms compared to those receiving unmodified saRNA. It underlines the fact that, despite being in relatively early development, research is gathering pace to enhance the efficacy and safety of saRNA vaccines. New pathways for innovative vaccine development are sure to open up as a result.
Working with saRNA – what you need to know
Although templates exist for saRNA success, branching out into a new field of therapies is still strewn with potential pitfalls for biomanufacturers. Accounting for four key elements of their design can help to guide the way.
Vector
The saRNA vector is crucial for ensuring the antigen’s effective delivery and expression. saRNA vectors typically include a large open reading frame that encodes the necessary machinery for RNA replication, adapted from viral sources. This setup enables the vaccine’s self-amplification – one of saRNA’s key benefits – but requires careful and considered design to prevent rising infection risk. The process mimics a viral infection in the host cells, enhancing both local and systemic immune responses – a feature that is fundamental to the vaccine’s efficacy.
Antigen
Selecting the relevant antigen is a key to saRNA vaccine creation. These can range from viral glycoproteins covering diseases transmitted via respiratory, insect, animal, and even bodily fluids to bacterial and parasitic antigens. Recent studies have shown promising results, including partial protection against bacterial infections in model systems and complete protection against parasitic reinfections in mice. Additionally, saRNA can be engineered to produce monoclonal antibodies, providing a novel method for passive vaccination.[3] Cancer vaccines are also a focus, with trials underway to test saRNA-based therapeutics that target specific neoantigens in solid tumours.
Delivery systems
Recent advancements have focused on encapsulating saRNA in lipid nanoparticles or cationic nanoemulsions to enhance cellular uptake and protect the RNA from degradation. Ongoing clinical trials are crucial for validating such delivery methods. Studies currently being conducted on SARS-CoV-2 vaccines are helping to confirm and expedite these validation processes, pointing to a bright future for saRNA.
Manufacturing
With antigens, vectors and delivery systems all accounted for, the final stage is manufacturing. Manufacturing saRNA involves in vitro transcription processes similar to those used for mRNA. They must, however, be optimised for longer RNA chains. TriLink’s CleanCap® technology, particularly the CleanCap® AU, is instrumental in this process, enabling efficient capping of saRNA that enhances its stability and in vivo activity. The result is rapid design and production cycles which can be as short as 14 days, pointing to their potential for combatting emerging infectious diseases and rolling out seasonal vaccines.
How picking the right partner can help
The potential of saRNA technology could give biomanufacturers unprecedented advantages in terms of development speed. But getting from theory to practice, particularly for such a novel therapeutic approach, can consume development times. This is why working with an industry expert – for example, in the area of RNA capping and manufacturing – is crucial for streamlining the process.
This is where TriLink comes in. TriLink’s innovative co-transcriptional capping method significantly enhances the efficiency of saRNA production. Their novel CleanCap® AU analog is tailored specifically for saRNA applications, facilitating the replication process critical for vaccine efficacy (download the technical guide to learn more). Their technology helps ensure that saRNA molecules are capped effectively, mimicking the natural structure of alphavirus RNA and streamlining their successful translation in the body.
The result enables higher capping efficiency, higher yields of functional saRNA and lower effective doses in vaccine formulations. It also provides enhanced vaccine potency while reducing overall manufacturing cost. And the one-pot synthesis approach of CleanCap® technology underpins a speedy manufacturing process, reducing the complexity and time required to produce each batch of saRNA.
TriLink and their CleanCap® AU analog are helping to futureproof biomanufacturers in an ever-changing pharmaceutical landscape. In additional to infectious disease vaccines, the coming wave of personalised cancer vaccines will benefit from the kinds of technology saRNA specialists are already using; the ability to quickly produce saRNA that can express multiple neoantigens specific to an individual patient’s tumour is invaluable. CleanCap® technology supports the development of these tailored vaccines by ensuring high-quality saRNA that can safely and effectively trigger a desired immune response.
Biomanufacturers need all the help they can get in the competitive and complex landscape of vaccine development. TriLink is determined to make each step of saRNA production – from synthesis to clinical application – as swift and smooth as possible. By significantly shortening the timeline from concept to clinic, innovative vaccine manufacturers can combine with TriLink expertise to confront the global health challenges of the future. Download the technical guide on this page to find out more.
[1] https://pubmed.ncbi.nlm.nih.gov/37586325/
[2] https://www.biorxiv.org/content/10.1101/2023.09.15.557994v1
[3] https://doi.org/10.1016/j.omtm.2020.06.011