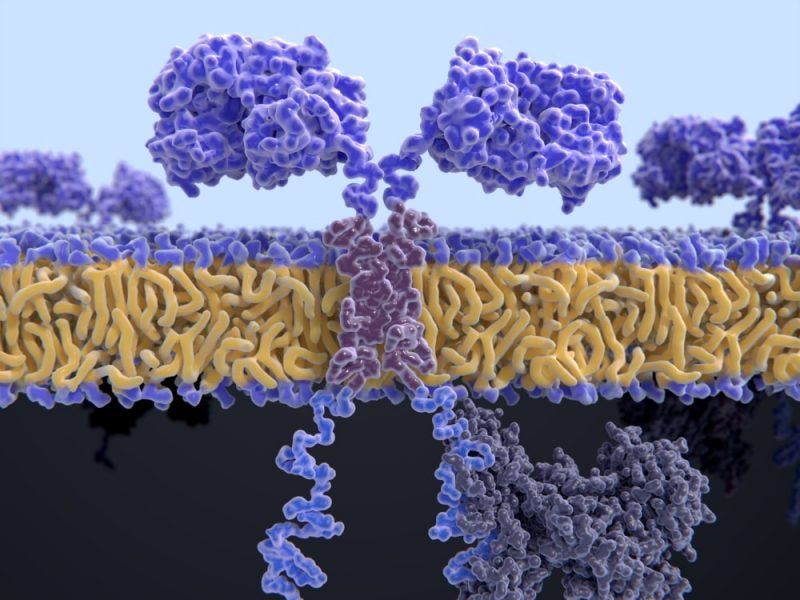
Chimeric antigen receptor T-cell (CAR-T) therapies represent an emerging frontier of personalised medicine for cancer. There are currently two CAR-T therapies currently on the market – Novartis’ Kymriahand Gilead’s Yescarta.
In this form of cell therapy, T cells are removed from a patient’s blood, genetically modified in a laboratory using a viral vector to produce CARs on their surface, which helps them to target antigens on tumour cells when they are infused back into the patient.
Despite the incredible breakthrough CAR-T therapies represent, there have been many issues related with their genuine ability to cure cancer. These complications are primarily the result of complex and expensive manufacturing processes, which drive up the costs of therapies- more than $400,000 per patient – thereby limiting the number of patients who can be treated with these therapies.
Boston-based engineering company Draper believes it has found a way to overcome these challenges related to autologous cell therapy approaches, which rely on viral vectors to deliver genes into the patient’s T cells. Among other techniques, Draper has developed a microfluidic electroporation platform replacing viral vectors with electricity; the company recently published a proof-of-concept study in the journal Lab on a Chip.
Draper’s head ofprecision medicine and cell bioprocessing Jenna Balestrini discusses why the current viral-vector, autologous approach to CAR-T manufacturing is inefficient, and how Draper’s new automated systems can overcome these challenges to enable CAR-T to reach its full potential as a cure for cancer.
Allie Nawrat: Two CAR-T therapies are now approved in various countries in the world, Yescarta and Kymriah. Can you explain the viral vector autologous manufacturing processes used for these and the major flaws of this approach?
How well do you really know your competitors?
Access the most comprehensive Company Profiles on the market, powered by GlobalData. Save hours of research. Gain competitive edge.
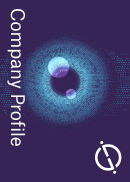
Thank you!
Your download email will arrive shortly
Not ready to buy yet? Download a free sample
We are confident about the unique quality of our Company Profiles. However, we want you to make the most beneficial decision for your business, so we offer a free sample that you can download by submitting the below form
By GlobalDataJenna Balestrini: Talking generally, the way CAR-T is developed currently is through an autologous approach.
What usually happens is you take cells from a patient; you then use a complicated, heavy biological approach to separate out the T cells: this involves magnetic beads and biological agents. From there you do a gene modification, meaning you activate them to prepare them for a virus; T cells aren’t necessarily [naturally] inducible to viral entry. Viruses are used [as a vector] as they have evolved to hijack host’s machinery to deliver genes of their interest into the host, so industry has effectively hijacked the hijackers to make them therapeutic delivery agents.
Separation and activation of T cells takes about 24 hours. Then virally inducing them takes another 48 hours [as it requires] introducing the genes into the genome and transforming them into CAR-T cells that can hunt down specific hematologic or blood cancers. You then expand them for a few days.
There’s around 100 steps to develop CAR-T therapies on average, and you have to do quality control at every single step. This means taking more material away from your starting population, so you need billions of cells for this to be an effective therapeutic; in fact, it’s three CAR-T cells to one cancer cell.
What that means for a patient is twofold. First, not a lot of patients qualify; paediatric patients, for example, don’t have enough starting material or maybe patients who have been through too many rounds of radiation therapy to have appropriate starting material. So that narrows the patient access window. Secondly, when you take cells out of the body [to create CAR-T therapies], the cells lose those important keys to keep them healthy and happy, meaning the T cells themselves become less good at attacking cancer.
AN: How can Draper’s approach reduce costs and other inefficiencies of the existing system?
JB: [It is usual] for biology to be behind the manufacturing technology. But in the case of CAR-T therapy, the biology is way more advanced than the manufacturing equipment. [In traditional approaches, you are] actually retrofitting equipment made for other lead processing applications and trying to force-fit them into making cell therapy, [which] is inefficient.
At Draper we’re trying to create fit-for-purpose devices. Instead of using these really old approaches to separate cells, activate them or virally transduce them, we’re trying to come up with new ways to use physics instead of biology to manipulate the T cells. Precision engineering [allows] you to tightly control the cells and the material that you’re trying to either introduce or integrate into those cells. You can also control their micro-environments to make sure they’re not having mechanical issues that are going to be problematic later.
This is takes the manufacturing process down from [roughly] a 22-day process to closer to 48 hours. You are not just saving money, you are also making a much higher quality product, which is better at treating the cancer.
AN: Could you explain precisely how Draper’s manufacturing approach, and specifically the microfluidic electroporation-based platform, works?
JB: We have four separate modules that address the different steps in the cell therapy making process: an acoustic separation platform, a viral transduction platform to accommodate for situations that need to continue to use viral vectors, an electroporation unit and an inline buffer exchange unit to reduce loss when changing media or washing cells.
The standard method right now to introduce the gene to T cells is viruses. [Although] viruses are efficient at delivering genes, there are limitations in the activation step and they are expensive. Also, the genes [needed] for next generation therapies are really big, and you can’t fit them all in a virus.
[With our electroporation approach], we wanted to address those issues and enable precision control of new biological tools by using a new way of getting genes into cells that doesn’t necessarily need activation and it’s an instantaneous process.To get genetic material into the nucleus of the cell and integrate [it] into the genome, there are two membranes – the outer cell membrane and the inner nuclear membrane – that you must bypass.
We looked to the field and saw that electroporation was one way that researchers were delivering genes. It delivers a voltage across the cell to shock them, which temporarily creates tiny pores across the two membranes. Through those holes, you gain entrance into the cells, and then we use a secondary electric theoretic guidance to guide the DNA into the cell. This works because DNA and RNA is charged, which you can manipulate to move it around.
One of the problems with electroporation is it delivers enormous voltages across cells in an uncontrolled way, which creates a huge amount of variability. [But] we leveraged knowledge about microfluidics internally [to overcome this challenge]; using microfluidics, you can manipulate fluids and create a tighter electric field to make sure the cells are not exposed to too much or too little material.
Incorporating our other technology, like acoustic waves for example, we can move in and out of different fluids, allowing us maximise the gene delivery without killing off our cells. That is revolutionary in terms of being able to create a lot of gene modified cells without having a ton of loss.
AN: At what stage of testing is Draper’s CAR-T manufacturing process?
JB: Each of our four modules are at different stages of development, but all of them have been evaluated by pharma partners. The way we classify development is stage one to four.
Stage one is bench top and alligator clips. Stage two is demo-ready, [where] we can take it to a pharma partner and demonstrate it using their therapeutic materials. Stage three is when the customer is using an alpha/beta prototype. Stage four is mass-manufactured and commercial-ready.
We are somewhere in stage two for most of them, and stage three for the viral vector technology. The electroporation unit is somewhere between stage two and three.
The idea is that you can use the modules standalone or you could connect them. Pharma pipelines are pretty diverse, so you want a lot of flexibility in the process, but you [also] want something that’s closed and automated. Our vision is to be able to accommodate a wide range of needs. We’re trying to create a system that will pace with what the market needs, we’re being forward thinking and introducing new tools that don’t exist to liberate the field.
We encourage the pharma companies to dream bigger, like you are not limited to the tools you have in front of you; we can help you we can build you better tools and drive innovation.
AN: CAR-T therapies are already beginning to revolutionise cancer treatment. How do you predict personalised medicine will transform the oncology space in the coming years?
JB: The two therapies on the market are for a very small contingency of people; two kinds of blood cancers that are age bracketed and only for patients who have gone through several rounds of first-line therapeutics.
The future is to get these therapies to patients earlier and for them possibly to be used as frontline therapeutics. I know that groups like Novartis are doing clinical trials to see if we can move the needle closer to first-line.
I think eventually there’s a chance that CAR-T therapies will be used as much or even more than therapies like chemotherapy and radiation. You’ll start to see the more complicated cancers, like solid tumours, be treated with cell therapies in conjunction with other therapeutics, like monoclonal antibodies and B1 inhibitors.
The very future could be off-the-shelf products. Right now, we’re highly dependent on patient material and autologous material, but a lot of people don’t have enough starting material or time to live for this to be appropriate. Instead you make a universal donor out of a cell and knock out the components of that cell using typically CRISPR gene editing to create a universal therapeutic for one type of cancer.
If you had told me five years ago there would be a cure for cancer on the market, I wouldn’t have believed you.