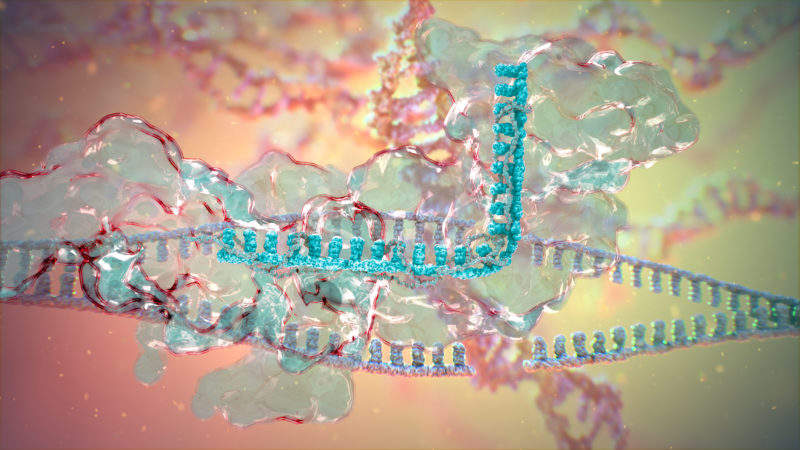
Given the ubiquity of CRISPR today, it’s hard to believe that the technology was little more than a blip on the biotech radar until a few years ago. While today’s understanding of CRISPR/Cas9 represents the sum of years of research since the CRISPR mechanism was discovered in the late 1980s, it was a seminal 2012 paper by professors Jennifer Doudna and Emmanuelle Charpentier – of University of California, Berkeley and Umeå University in Sweden, respectively – that provided the definitive illustration of CRISPR/Cas 9’s potential as a gene editing tool that could redefine the scope of what’s possible in drug development and other biotech-related fields.
Since then, the adoption of CRISPR has spread rapidly, not only among academic labs and specialist biotechs such as CRISPR Therapeutics and Editas Medicine, but also large pharma firms, with the likes of Merck, Novartis and Bayer investing in CRISPR/Cas9 tools for clinical research. According to a 2017 report by Grand View Research, the CRISPR genes market is set to reach a value of over $4bn by 2025.
“There is probably no large biotech or pharma company out there anymore, who have their own R&D, that are not using CRISPR,” said CRISPR Therapeutics CEO Dr Rodger Novak at a Labiotech Refresh biotech conference in 2016. “They are all using CRISPR in their labs.”
So what is driving the excitement about CRISPR/Cas9 in the biotech space, and how is the technology impacting drug development?
CRISPR: how does it work?
CRISPR is an abbreviation of ‘clustered regularly interspaced short palindromic repeats’, which are repetitive patterns found in the DNA of bacteria and archaea. This pattern, as has been gradually elucidated by various labs over the last decade, allows microbes to copy and incorporate the DNA segments of invading viruses into their genome between CRISPR’s short DNA repeats. This gives the bacteria the blueprint it needs to ‘remember’ the DNA of future viral invaders, and then guide the clam-shaped Cas9 (CRISPR-associated) enzyme to the virus to cut it up and disable it.
In their 2012 paper, Doudna and Charpentier were the first scientists to describe how to use this naturally-occurring CRISPR/Cas9 mechanism to cut any DNA strand in a test tube, opening up the possibility to use it as a highly precise gene editor. This discovery was followed in 2013 by work from teams including Feng Zhang and George Church of genomic research centre the Broad Institute, who demonstrated how CRISPR/Cas9 could be used to edit genomes in more complex human cells.
In human cells, CRISPR/Cas9 gene editing works by transcribing CRISPR ‘spacer’ sequences into RNA sequences, which are capable of guiding the Cas9 ‘scissor’ enzyme to precisely cut the intended DNA. This mechanism opens up a host of options for clinical researchers. After the cut, eukaryotic cells work to repair the cut DNA using any available DNA, so by simultaneously injecting a new gene, it can be incorporated into the repaired DNA sequence, completing the edit. Alternatively, shutting off the gene with a cut (or using a modified Cas9 enzyme to switch the gene on and off without cutting it) allows researchers to study the gene’s function and how it may relate to human disease.
An edge over the competition
CRISPR is far from the only horse in the stable when it comes to gene editing; other mechanisms have long been in use by genome editing scientists, from zinc finger nucleases (ZFNs) and transcription activator-like effector nucleases (TALENs) to recombinant adeno-associated virus (rAAV). While there is still a fair amount of debate over the comparative efficacy of these methods, CRISPR has quickly become predominant in the space, with around 74% of gene editing research programmes now employing CRISPR over its rivals, according to data from Informa Pharma Intelligence.
What gives CRISPR the edge? The quick answer is the tool’s relative simplicity and scalability. As CRISPR/Cas9 can be applied directly in embryo, the process is much faster and less expensive than other genome editing technologies, as well as not being reliant on separate cleaving enzymes to cut DNA.
“[CRISPRs] can easily be matched with tailor-made ‘guide’ RNA (gRNA) sequences designed to lead them to their DNA targets,” notes the Broad Institute on its website. “Tens of thousands of such gRNA sequences have already been created and are available to the research community. CRISPR/Cas9 can also be used to target multiple genes simultaneously, which is another advantage that sets it apart from other gene-editing tools.”
A powerful tool for drug discovery
While the prospect of using CRISPR-derived genetic therapies to more effectively treat genetic disorders or cancer is an exciting one, this research is years away from maturation, with the first early-stage clinical trials only just starting to emerge. In the here and now, CRISPR has become invaluable as a drug discovery tool for laboratories and biotech firms.
“The targets we’re finding with CRISPR/Cas9 are going to guide the drugs coming out in the 2020s,” enthused Dr Jon Moore, chief scientific officer at UK-based genomics company Horizon Discovery, at an event hosted by the firm in London in 2016.
CRISPR allows researchers to activate or inhibit genes with unprecedented ease, helping them to identify those that cause (or prevent) disease, offering better targets for therapeutic intervention. CRISPR’s relatively high accuracy in terms of cleaving DNA correctly and avoiding off-target effects has made it easier to run large-scale, meaningful tests on genetic function.
CRISPR significantly simplifies the process of identifying drug targets through functional genomic screens, as well as developing useful disease models to test drug efficacy. If drug discovery is an endlessly complex treasure hunt, CRISPR is a metal detector, and with 1,185 CRISPR-related scientific publications in 2015 alone, the research community is adopting the tool at a blistering pace.
A recent example comes from the Cold Spring Harbor Laboratory (CSHL) in New York, where scientists used a CRISPR-based system to screen large numbers of genes and assess their impact on cancer cell survival. The team discovered two enzymes, LKB1 and salt-inducible kinase, that are central to the survival of certain acute myeloid leukaemia (AML) cells, which had previously not been linked to the disease’s progression. Inhibiting these targets has opened a new route to blocking the growth of AML cells.
“We just let the cancer cells tell us what types of genes they need in order to grow,” said CSHL associate professor Christopher Vakoc.
Longer-term prospects for CRISPR therapies
In the longer term, labs are working to bring gene editing to bear on innovative new therapies that could have profound implications for the treatment of genetic diseases and cancers. Although these are a much more distant prospect than the drug discovery tools being used today, dedicated research teams are working towards clinical trials.
CRISPR Therapeutics and its partner Vertex Pharmaceuticals are planning Europe’s first CRISPR/Cas9 clinical trial for the treatment of inherited blood disorder beta thalassaemia using gene editing therapy candidate CTX001, with another trial of CTX001 in sickle cell disease planned in the US. The partners have already presented pre-clinical data suggesting that over 80% of human haematopoietic stem cells could be edited consistently, and the therapy, which took place ex vivo before being infused into mice models, prompted increased production of foetal haemoglobin.
Elsewhere, promising advances have been made at Poland’s Institute of Bioorganic Chemistry using CRISPR gene editing as a potential treatment for Huntington’s disease, which is currently incurable, while in China, scientists at Sichuan University injected CRISPR-modified white blood cells into a patient with metastatic lung cancer back in 2016, the world’s first (primarily safety-focused) trial of CRISPR gene editing in a human.
While the therapeutic potential is obvious, there are a number of obstacles that remain before CRISPR gene editing can break into the mainstream, including a somewhat acrimonious patent dispute between the key figures in the mechanism’s discovery and development, as well as societal controversy over the bioethical implications of CRISPR-enabled ‘germline editing’, or heritable gene editing that would affect future generations, raising the spectre of ‘designer babies’ and the grave risk of inherited effects if DNA cleaving had unintended genetic casualties.
Beyond these, efforts are ongoing to refine CRISPR/Cas9’s reliability and accuracy, with researchers at MIT and Harvard University last year announcing the development of a tweaked form of CRISPR capable of base editing – the ability to modify a single DNA base without breaking the DNA strand – offering the chance to cleanly repair disease-causing point mutations.
CRISPR will need these improvements if it’s to remain on course as the predominant gene editing tool, as competition from other technologies such as ZFNs, TALENs and rAAV is strong, as demonstrated by Gilead’s recently-announced partnership with Sangamo Therapeutics to use ZFNs as a gene-editing enhancement to its cell-based immunotherapy portfolio.
“I don’t even think CRISPR/Cas9 is going to be the end-all,” said Informa Pharma Intelligence principal analyst Amanda Micklus in an October 2017 interview with MedCity News. “I think that as we move forward, as more discoveries are made, there’s going to be more tools added to the gene editing toolbox and we’ll have to see how some of these candidates play out.”
It’s clear that as an enabler of drug discovery and a route towards revolutionary new treatments, gene editing is here to stay. CRISPR, with its user-friendliness and suitability for large-scale genetic screening, has edged to the front of the pack as the tool of choice, but whether it’s the future or simply a bridge to something better remains to be seen. Whatever happens, with the ability to edit the very building blocks of life at the world’s fingertips, there’s no going back now.